Microwave Power Amplifiers for Broadband Applications
click here to download the full copy .png)
Amplifiers are one of the most basic electrical elements in any electronic system. Broadband microwave power amplifiers are used in test and measurement applications when increased RF power is necessary for overcoming cable and system losses in ATE or to radiate a device-under-test (DUT) with an intense electromagnetic field as may be found in EMI/EMC applications.
The specific characteristics of a given amplifier can be as varied as the applications. Nevertheless, there are common requirements for nearly all amplifiers including frequency range, gain and gain flatness, power output, linearity, noise figure, VSWR and stability. Often, there are design trade-offs required to optimize any one parameter over another and performance compromises are usually necessary for an amplifier that may be used in a general purpose testing application.
Broadband amplifiers, covering a decade or more in bandwidth are difficult to achieve, especially at higher power levels. The product of power and bandwidth is fixed for a specific amplifying device. That is, the broader the bandwidth, the less the output power. There are numerous techniques for designing microwave power amplifiers. These may be split between tube and solid-state technologies.
RF/Microwave Instrumentation amplifiers are typically characterized by broadband frequency range, high gain with relatively flat frequency response, low noise and wide linear range. Instrumentation amplifiers can be either solid-state power amplifiers (SSPA) or traveling wave tube amplifiers (TWTA) depending on the power level. TWTAs provide the highest power levels available, but solid-state power amplifiers are continually increasing in capability, and the advantages that solid-state technology offers over vacuum tube technology are significant.
Power requirements for greater than 100 Watts are typically satisfied with tube-based designs. Tube amplifiers, such as TWTAs, require a high voltage power supply, typically require warm-up time, have significant aging related issues and are relatively expensive. Also, solid-state amplifiers exhibit little AM-PM conversion to their rated 1 dB compression point. TWTAs must typically be backed off 16 dB or more from their rated saturated power level before achieving minimal AM-PM.
Table 1 below summaries a performance comparison between solid-state Parallel-MMIC amplifiers and TWTA’s.
Table 1) Solid-State Amplifier Technology versus TWTA Technology
Parameter |
Solid-State Power Amplifier |
TWTA |
Bandwidth Capability |
Decade or more |
One to Two Octaves |
Noise Figure |
< 10 dB |
> 25 dB |
Gain |
Medium |
High |
Gain Flatness |
± 3 dB |
± 8 dB |
Harmonics at Psat |
< 20 dBc |
0 to -6 dBc |
Phase Noise and Thermal Noise Power |
Low |
High |
Load Mismatch Tolerance |
Good |
Poor |
Reliability |
> 100k Hours |
< 10k Hours |
Test and measurement applications requiring higher power, greater than 1 Watt, were traditionally dominated by TWT amplifiers. These applications can now take advantage of all the performance advantages of solid-state implementation, including higher reliability, safe low voltage operation, longer life, low thermal noise, improved linearity and no warm-up time. Solid-State amplifiers offer over a decade of bandwidth, surpassing current tube technology by a factor of two or more.
One approach to achieving higher power over broad frequency range is to switch between multiple narrowband amplifiers, with degradation to the overall linearity and gain flatness. Switching is never ideal. Mechanical switches, while quite linear and relatively low loss, having speed limitations and subject to failure after repeated switching cycles. Solid-state switches overcome the speed issue but are not nearly so linear or low loss. Both the signal fidelity and loss issues limit the usefulness of solid-state switches for high power microwave amplifiers. It should also be noted that this approach only works with CW or narrowband signals, and is not a solution for broadband signals such as UWB radar.
A topology often favored for generating modest amounts of microwave power is to combine the outputs of several relatively low power amplifiers. The individual amplifiers usually have a distributed or traveling wave topology. Distributed amplifier designs have been widely used to achieve chip level broadband solid-state amplifiers with a decade and greater bandwidth. This amplifier approach is often fabricated using MMIC (Monolithic Microwave Integrated Circuit) techniques, and has been optimized to the point where single MMIC amplifiers can provide up to nearly 1 Watt of saturated output power.
New techniques employing spatial combining enable high power and flat gain over a broad bandwidth. Spatial combining can be used to sum a much larger number of amplifiers over a decade or greater frequency range than would be practical using conventional planar circuit techniques. These broadband microwave power amplifiers exhibit exceptional performance at prices typically less that of multiple narrowband amplifiers.
Spanawave provides solid-state RF/microwave instrumentation amplifiers for applications such as laboratory instrumentation, EMC test, defense EW/radar test and commercial communications test. See Figure 1.
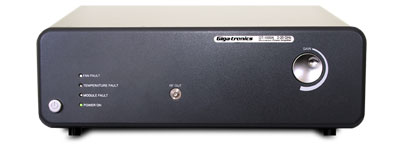
Figure 1) Spanawave/Giga-tronics GT-1000A Solid-State Microwave Power Amplifier
The Spanawave/Giga-tronics solid-state amplifiers use GaAs parallel MMIC technology in a spatially combined amplifier core. Spatial power combining, developed and patented in the Spatium™ implementation by CAP Wireless of Newbury Park, California, is a unique structure providing an optimum method of achieving multiple device power combining. See Figure 2. This approach weds advantages of the traveling wave MMIC amplifiers with the high efficiency of spatial combining.
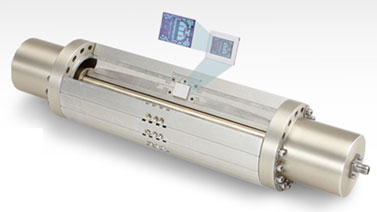
Figure 2) Spatium™ Amplifier Core
The uniformity of the MMICs and the intrinsic symmetry of the structure enable nearly identical phase and amplitude through all amplification channels, resulting in high combining efficiency, high gain and flat frequency response. That combined with the very broad frequency range provides outstanding pulse performance. Also, because of the high number of combined devices, the RMS phase noise is less than that of a single device and significantly lower that from a comparable TWTA.
Higher power is required when signal purity and very linear amplification are critical for analog and digital amplitude modulated signals or any signal with high peak to average ratio. If the peak to average ratio, or crest factors are high in the signals that are to be amplified, then you need to ensure that the amplifier has enough head room. This requires operating the amplifier in the linear range backed off below the saturated output power.
TWT amplifiers have a power rating defined by their peak power output capacity and must be operated substantially (typically 6 dB or more) below their rated saturated output power for linear performance. Solid-state amplifiers, on the other hand, have power ratings defined by their one or two dB gain compression point and can be operated at or near their rated one dB compression level to achieve linear performance. This means, for example, that a solid-state amplifier rated at 10 W P1dB will offer equal or better linear performance compared to a TWTA rated at 40 or more watts of saturated power. In terms of linearity, TWTAs have a very soft saturation curve, starting to saturate well before reaching their peak power. In comparison, solid-state GaAs parallel-MMIC amplifiers have a very sharp saturation curve, staying linear until very close to reaching peak power.
Solid-state parallel-MMIC amplifiers have very broad frequency ranges with exceptional gain flatness of nominally ± 3 dB. Broadband TWTAs have much coarser gain flatness on the order of ± 8 dB. TWTAs typically have very high gain, typically 50 to 60 dB, while solid-state amplifiers are typically 25 to 35 dB. There are some applications where the higher TWTA gain is beneficial, such as in many radar applications, but it can also be problematic. It can result in higher noise levels or it can require attenuating the output of signal generators or other signal sources where 0 dBm to +10 dBm output levels are common. Solid-state amplifiers have an inherently low noise figure, typically no more than 8 to 10 dB. TWTAs often have noise figures ranging from 30 to 35 dB, potentially limiting the dynamic range of many measurements.
Spanawave/Giga-tronics amplifiers use solid-state devices, which inherently provide high reliability and are inherently mechanically rugged. TWTAs, on the other hand, are subject to damage by abuse or shock because they are highly mechanically tuned, vacuum-encapsulated-by-glass devices. Solid-State parallel-MMIC amplifier technology takes advantage of available GaAs microwave semiconductor power device technology by combining multiples of devices to achieve higher output powers. Because this device technology operates from safe low (< 28 VDC) supply voltages, rather than the high (> 1 kV) supply voltages typically associated with TWTAs, it can operate from readily available, lower cost power supplies. Power supply design complexity is reduced and the power supply mean time between failures (MTBF) is therefore slightly better than for the TWTA power supplies.
Conclusion
In conclusion, the Spanawave/Giga-tronics GT-1000A Microwave Power Amplifier designed using a Spatium™ Amplifier Core is a high-performance RF/Microwave Instrumentation amplifier for bench top and ATE system applications. Providing solid-state reliability and unique electrical performance, the GT-1000A is ideal for applications such as laboratory instrumentation, EMC test, defense EW/radar test and commercial communications test.
|